Resonance Effect
In organic chemistry, the resonance effect is a phenomenon where a substituent on a molecule influences the electron density distribution through π-electron delocalization. This effect is crucial for understanding the chemical behavior of molecules, particularly how substituents affect molecule stability, reactivity, and acidity/basicity.
1.0Mechanism of the Resonance Effect
The resonance effect occurs when electrons within a molecule are delocalized through π bonds or between a π bond and a lone pair of electrons on an adjacent atom. This electron delocalization can either stabilize or destabilize the molecule depending on the nature of the substituent:
Electron-Donating Groups (EDGs):
- These groups push electron density through the π system, increasing electron density on other parts of the molecule. This is typically done by substituents that have lone pairs of electrons they can share via resonance.
- Common examples include groups like -OH (hydroxyl), -OCH3 (methoxy), and -NH2 (amino). These groups increase the electron density through their lone pairs, making the system more nucleophilic.
Electron-Withdrawing Groups (EWGs):
- These groups pull electron density towards themselves via resonance, decreasing electron density on other parts of the molecule. They typically contain electronegative atoms or groups that are capable of accepting electron density.
- Examples include - CHO (aldehyde), NO2 (nitro), -CN (cyano), and -COOH (carboxylic acid). These groups withdraw electron density, making the system more electrophilic and often more acidic.
Here is visual representation of Electron-Donating (-OH, hydroxyl) and Electron-Withdrawing (CHO,aldehyde) Group
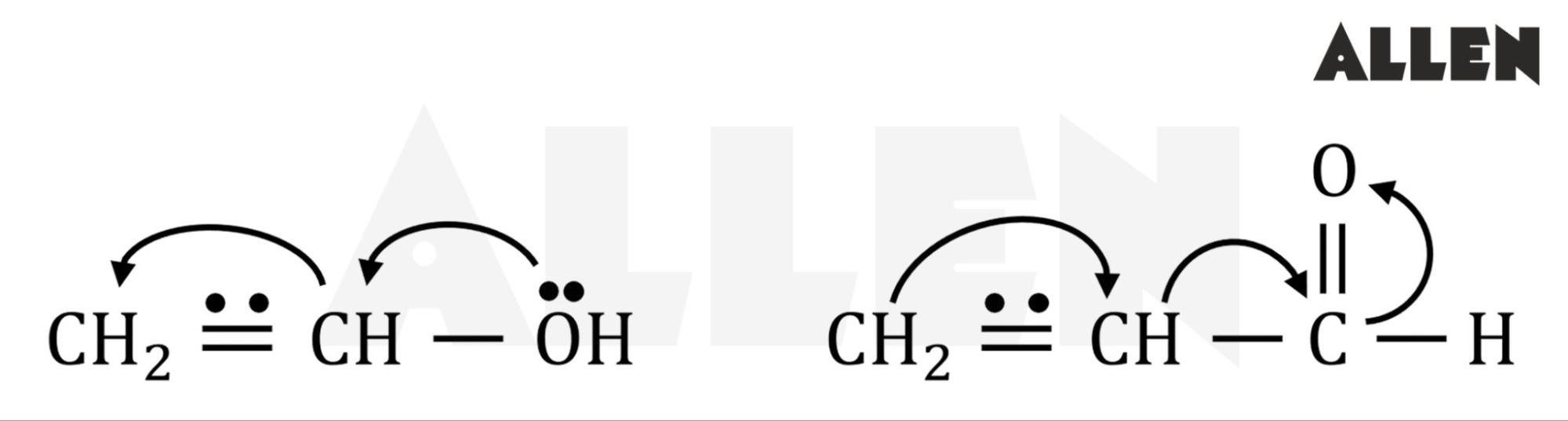
2.0Resonance Structure
The concept of resonance is fundamental in organic chemistry as it explains the stability of many organic compounds, particularly those containing aromatic rings. For example, the benzene ring remains unusually stable not because of single or double bonds between carbon atoms, but due to the delocalization of electrons described by the resonance effect. This delocalization leads to structures called resonance forms or contributors, which are hypothetical and do not exist individually but combine to give a more accurate description of the actual molecule. The actual structure of a molecule exhibiting resonance is a hybrid of these forms, showing a more delocalized electronic structure than any individual contributing form.
Resonance structures in Benzene:
In benzene, resonance involves the delocalization of six pi electrons across the six carbon atoms, creating equal bond lengths and enhanced stability, as depicted by a hexagonal ring with a circle indicating evenly distributed electrons.
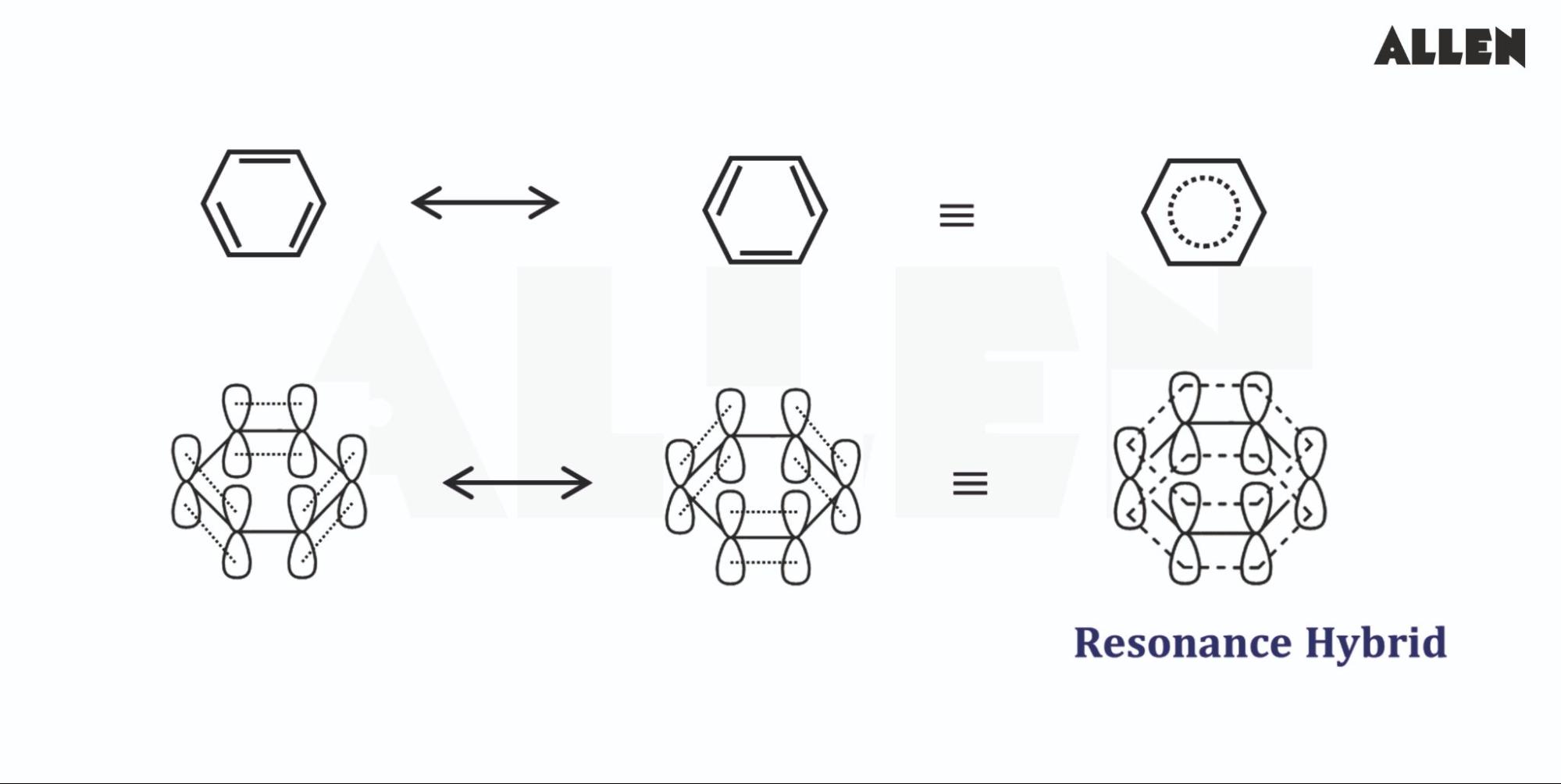
3.0Types of Resonance Effect
There are two primary types of resonance effects, categorized based on the nature and direction of electron movement:
- Positive Resonance or Mesomeric Effect (R+ or M+):
This effect occurs when electrons are donated or pushed towards an electron-deficient area within the molecule through π-bonds or from a lone pair on an atom adjacent to the π-system. Molecules or groups that can donate electrons via resonance stabilize cationic centers adjacent to them and are often termed electron-donating groups.
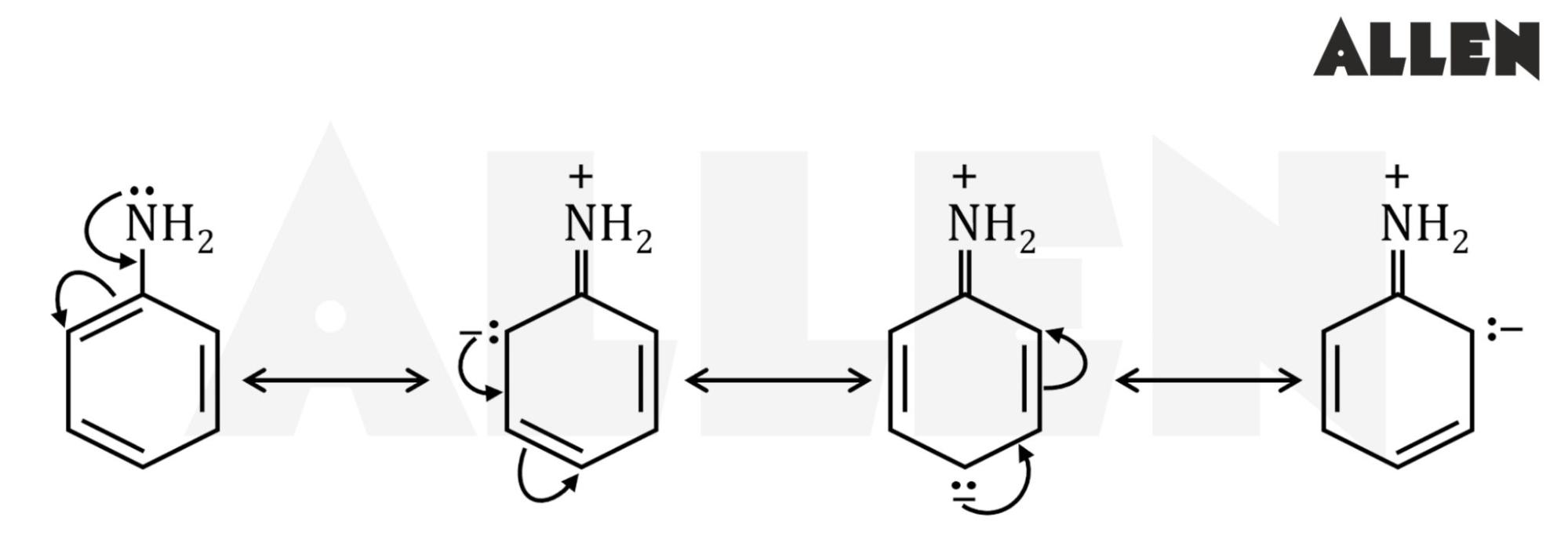
- Negative Resonance or Mesomeric Effect (R- or M-):
In this effect, electrons are pulled away from an area of the molecule towards a more electronegative atom or group, which can accept electrons. This is typically seen in systems where an atom within a π-system is electronegative and capable of drawing density towards itself. Groups that exhibit this behavior are known as electron-withdrawing groups and they stabilize anionic centers adjacent to them through resonance.
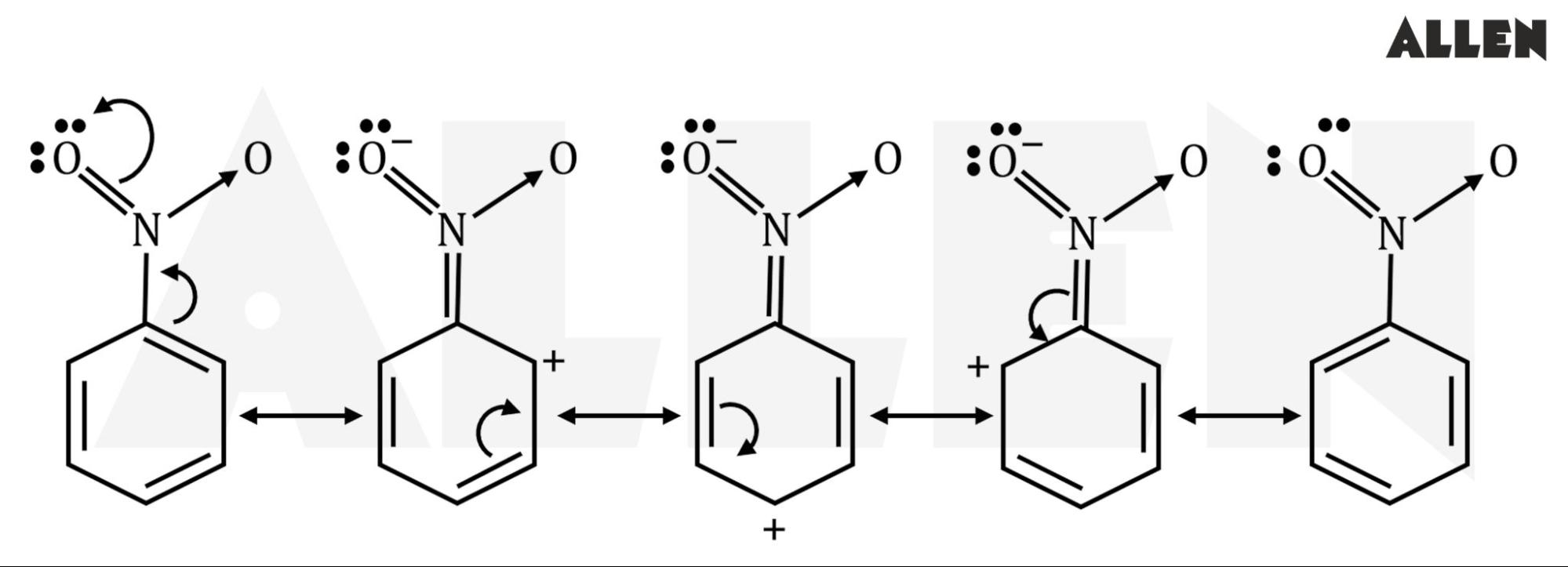
4.0Applications of the Resonance Effect
- Stability:
The resonance effect can stabilize certain intermediates and transition states in chemical reactions. For instance, carbocations adjacent to electron-donating groups are stabilized due to the additional electron density provided via resonance.
- Stability of Carbocations: Carbocations that can delocalize their positive charge through resonance with a double bond exhibit increased stability. An example is the allylic carbocation, which is more stable than a simple alkyl cation due to its ability to form resonance structures. In these structures, the positive charge is distributed over several atoms, thanks to the delocalization of π-electrons from conjugated double bonds. The greater the number of effective resonance structures, the higher the stability of the carbocation.
- Stability of Carbanions: The stability of carbanions is enhanced when they are adjacent to double bonds or within an aromatic ring. This stability boost arises from the resonance stabilization, where the negative charge can be delocalized across multiple atoms. For example, the benzyl carbanion is more stable than the ethyl carbanion because the negative charge in the benzyl carbanion is dispersed over additional carbon atoms, thanks to the aromatic ring’s resonance capabilities. Thus, larger resonance structures generally indicate greater stability.
- Stability of Free Radicals: Free radicals experience stability variations based on the delocalization of their unpaired electrons. Simple alkyl radicals are less stable compared to allylic and benzylic radicals, where the unpaired electron is delocalized across a π-system. This depolarization, or distribution of the electron density across multiple atoms, significantly enhances the stability of these radicals.
- Reactivity:
Molecules' reactivity can be altered by substituents through the resonance effect. Electron-donating groups can enhance the nucleophilicity of a molecule, making it more reactive towards electrophiles. Conversely, electron-withdrawing groups increase the electrophilicity of a molecule, making it more reactive towards nucleophiles.
- Acidity and Basicity:
The presence of electron-withdrawing groups can increase the acidity of a molecule by stabilizing the negative charge on a conjugate base through resonance. For example, the acidity of carboxylic acids is significantly enhanced by the presence of a -COOH group, which can stabilize the conjugate base (-COO⁻) through resonance. Similarly, electron-donating groups can decrease acidity by destabilizing the conjugate base due to increased electron density.
Table of Contents
- 1.0Mechanism of the Resonance Effect
- 2.0Resonance Structure
- 3.0Types of Resonance Effect
- 4.0Applications of the Resonance Effect
Frequently Asked Questions
The resonance effect refers to the delocalization of electrons in a molecule that contains conjugated bonds. This effect allows electrons to be spread over several adjacent atoms, providing the molecule with greater stability.
Resonance stabilizes a molecule by distributing electron density across different atoms and bonds. This distribution reduces the electron density on any single atom, lowering the potential for charge- or electron-based reactivity and thus stabilizing the molecule.
Common examples include benzene, nitrate ions (NO₃⁻), and carboxylate ions (RCOO⁻), where the double bonds and lone electrons are delocalized over the structure.
Yes, resonance can influence physical properties such as melting and boiling points, as well as chemical properties like acidity and basicity. For instance, the resonance in carboxylic acids increases their acidity by stabilizing the conjugate base.
Join ALLEN!
(Session 2024 - 25)