Atomic Structure
Atomic structure definition involves a fundamental concept in Chemistry and Physics, providing insight into the behavior and interaction of matter at the smallest scale. This article ensures that whoever seeks detailed information about atomic models, bohr atomic model etc., finds all the information here.
1.0What is Atomic Structure?
The concept of atoms as fundamental building blocks of matter dates back to early Indian and Greek philosophers around 400 B.C., who theorized that continuous subdivision of matter would eventually reveal indivisible atoms. The term "atom" originates from the Greek "a-tomio," meaning "uncuttable" or "indivisible." These early theories were speculative and not experimentally verifiable, lying dormant until the 19th century.
John Dalton introduced the first scientific atomic theory in 1808, describing atoms as the smallest units of matter and explaining key chemical laws.
However, his theory didn't explain electrical phenomena.
Later, 19th and early 20th-century experiments discovered subatomic particles—electrons, protons, and neutrons—refining our understanding of atomic structure beyond Dalton's indivisible atoms.
Electron: The electron was the first subatomic particle discovered. In 1897, J.J. Thomson through Cathod ray Experiment, which demonstrated that cathode rays were streams of negatively charged particles, later named electrons.
He showed that electrons are much smaller than atoms and carry a negative charge.
Proton: The discovery of the proton is credited to Ernest Rutherford. In 1919, through his experiments with nitrogen and alpha particles, Rutherford identified a positively charged particle, which he named the proton. He established that protons are located in the nucleus of an atom and have a positive charge that balances the negative charge of electrons.
Neutron: The neutron was the last of the three main subatomic particles to be discovered. In 1932, James Chadwick conducted experiments bombarding beryllium with alpha particles, which emitted a new type of radiation that he deduced was composed of neutral particles, or neutrons. Neutrons reside in the nucleus alongside protons and have no electric charge, contributing to the atom's mass without affecting its charge.
So basically atoms are made up essentially, of three fundamental particles, which differ in mass and electric charge as follows:
Property | Electron | Proton | Neutron |
Symbol | e or e⊝ | P or P⊕ | n |
Approximate relative mass | 1/1836 | 1 | 1 |
Approximate relative charge | -1 | +1 | 0 |
Mass in kg | 9.10939 × 10–31 | 1.67262 × 10–27 | 1.67493 × 10–27 |
Mass in amu | 0.00054 | 1.00727 | 1.00867 |
Actual charge/C | –1.6022 × 10–19 | +1.6022 × 10–19 | 0 |
2.0Atomic Models
Atomic models are theoretical frameworks used to describe the structure, behavior, and other properties of atoms in a way that explains both their physical and chemical properties. Over the years, these models have evolved significantly as scientists have gained new insights through experimentation and theoretical research. Here’s an overview of the key atomic models that have been developed:
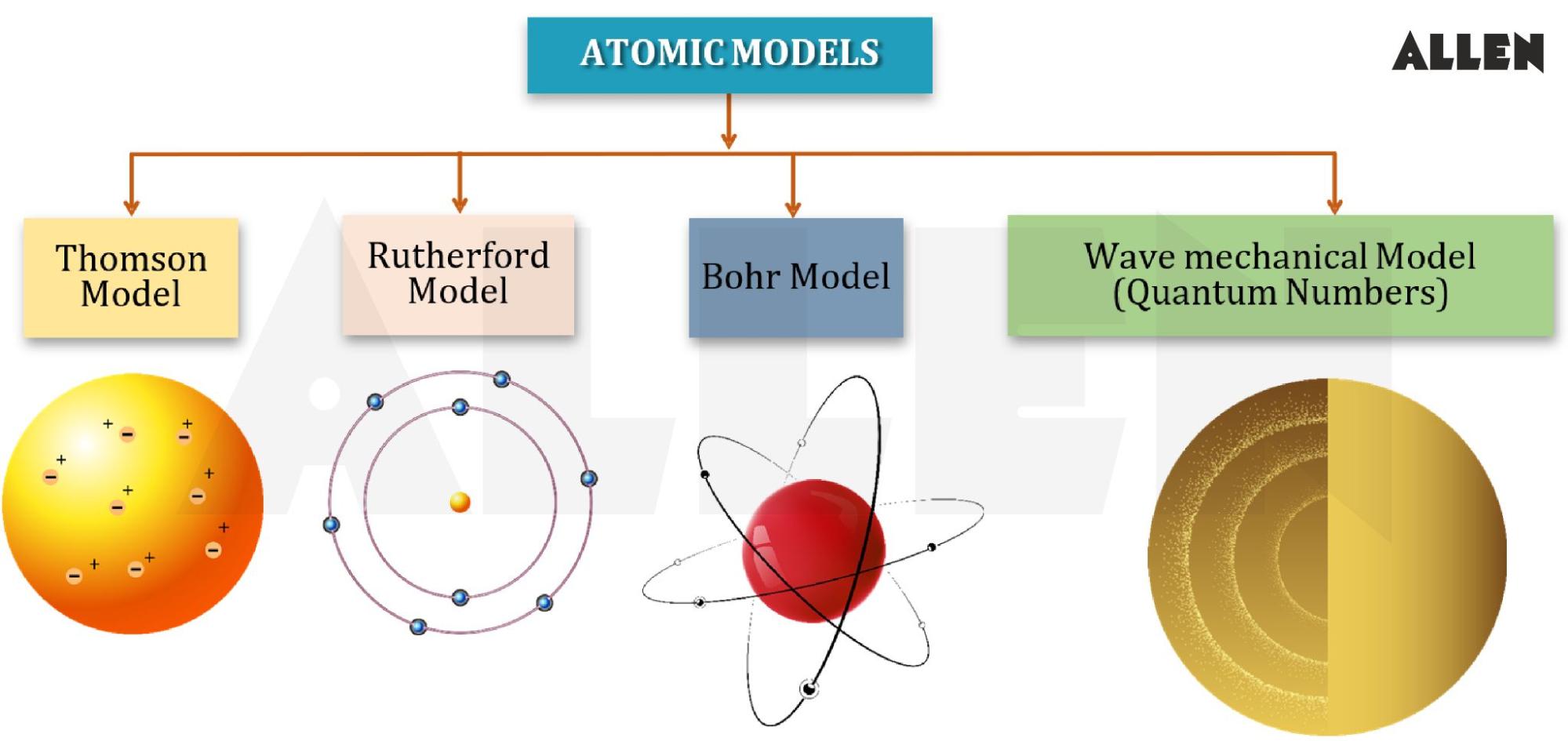
Dalton's Atomic Model (1808): John Dalton described atoms as solid, indivisible spheres, making up different elements. This model explained chemical reactions in terms of the rearrangement of these unbreakable atoms.
Dalton's theory explained:
- Law of conservation of mass
- Law of constant composition
- Law of multiple proportions
Theory limitations:
- Couldn't explain electricity generation in materials like glass or ebonite when rubbed.
- Suggested atoms have more complex structures than previously thought.
- Led to the discovery of subatomic particles.
Thomson's Plum Pudding Model (1898)
J.J. Thomson proposed that an atom is a sphere of positive charge with negatively charged electrons embedded within it, much like plums in a pudding. This model came after the discovery of the electron but before the nucleus was discovered.
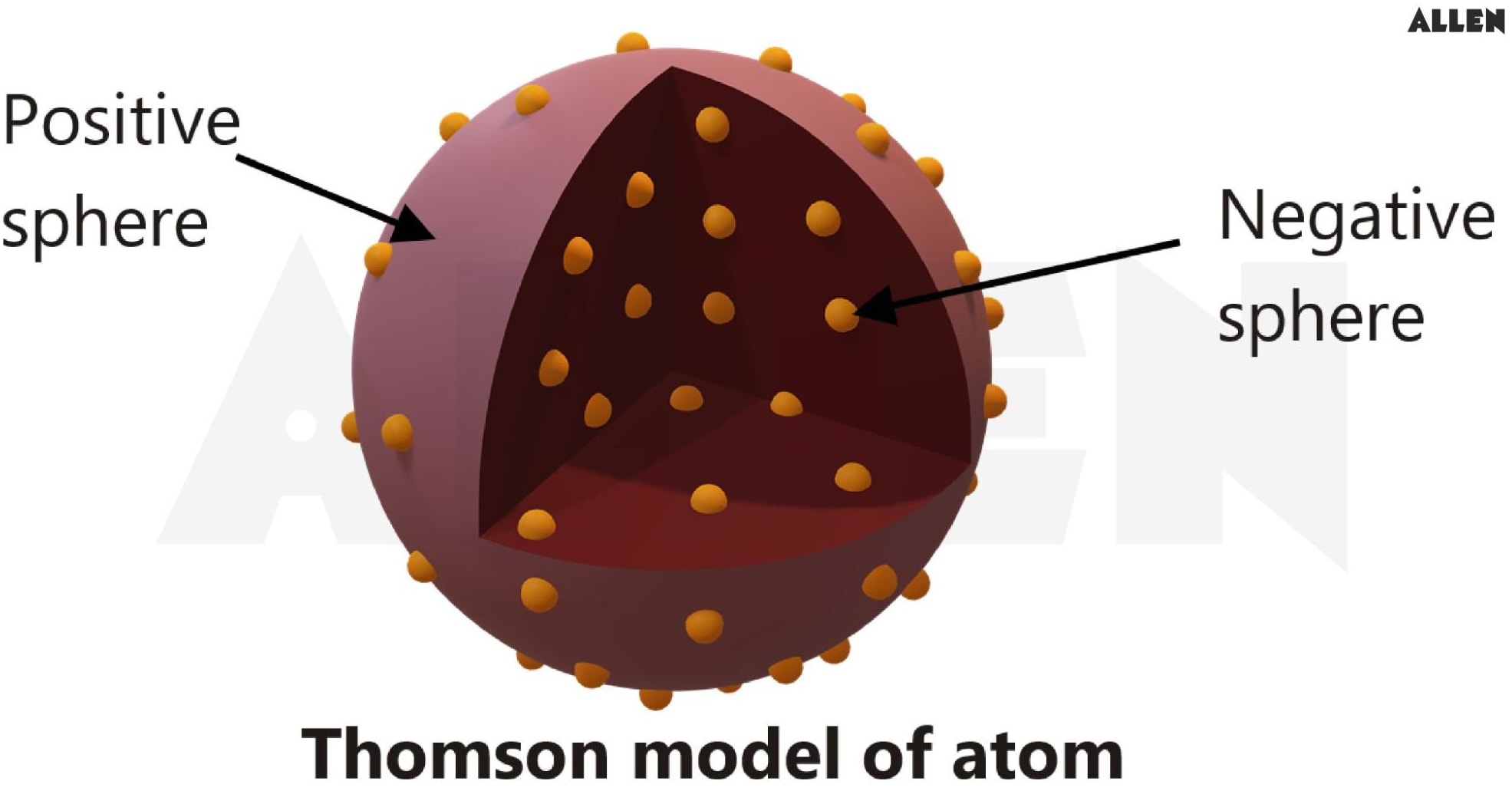
Rutherford's Nuclear Model (1911)
Rutherford's gold foil experiment involved shooting high-energy alpha particles at a thin gold foil and observing their behavior using a fluorescent screen. The unexpected results led to significant conclusions about the atomic structure:
Experiment Setup: High-energy alpha particles from a radioactive source were aimed at a thin (about 100 nm) gold foil surrounded by a circular fluorescent screen.
Observations:
- Most alpha particles passed through the foil without any deflection.
- A small fraction were deflected at slight angles.
- A very few (about 1 in 20,000) bounced back, nearly 180°.
Conclusions:
- The atom is mostly empty space, as most alpha particles went through undeflected.
- The presence of a small, dense, positively charged nucleus in the atom caused the deflection of some alpha particles.
- The nucleus is tiny compared to the atom's size, akin to a cricket ball's size versus a 5 km radius if comparing the nucleus to the atom.
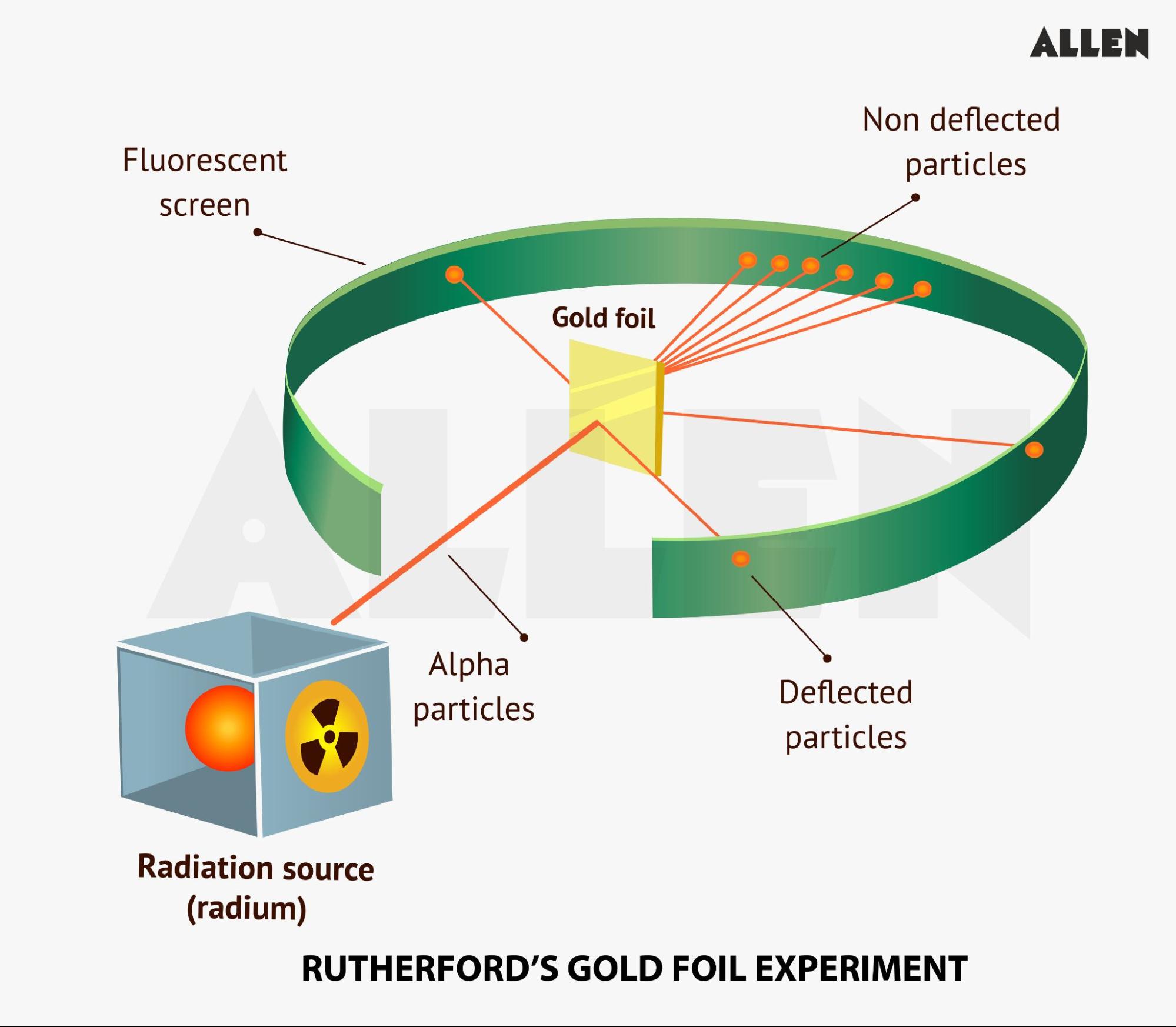
Drawbacks of Rutherford model:
The Rutherford model doesn't explain atom stability, lacks details on electron orbits, doesn't address how nuclei stay intact despite repulsive forces among protons, and can't account for the discrete lines in atomic spectra.
3.0Development of Leading Model of Bohr
Historical observations from studies on how radiation interacts with matter have significantly enhanced our understanding of atomic and molecular structures. Neils Bohr leveraged these insights to refine Rutherford's atomic model.
Two developments instrumental in shaping Bohr's atomic model were:
1. Dual Nature of Electromagnetic Radiation: This concept acknowledges that electromagnetic radiation exhibits both wave-like (Interference, Diffraction, Polarization) and particle-like properties (Photoelectric Effect, Black body radiation, Atomic spectra), embodying the principles of wave-particle duality.
2. Atomic Spectra Observations: Experimental findings related to atomic spectra necessitated the adoption of quantized electronic energy levels within atoms to explain the discrete spectral lines, aligning with the concept of energy quantization.
4.0Bohr's Atomic Model
Bohr's model for the hydrogen atom includes these core principles:
Niels Bohr's atomic model, proposed in 1913, was a significant development in understanding the structure of atoms. Here are the important points of Bohr's model:
1. Quantized Electron Orbits: Bohr proposed that electrons orbit the nucleus of an atom in specific quantized orbits. These orbits have discrete energy levels, and electrons can only occupy certain allowed orbits. This concept introduced the idea of quantization in atomic physics.
2. Fixed Orbits: In Bohr's model, electrons are assumed to orbit the nucleus in fixed circular paths without radiating energy. These orbits are sometimes referred to as "stationary states" or "energy levels." Electrons can move between these orbits by absorbing or emitting energy in discrete amounts, corresponding to the energy difference between the orbits.
3. Angular Momentum Quantization: Bohr postulated that the angular momentum of an electron in these orbits is quantized, meaning it can only take on certain discrete values. This quantization is expressed by the equation:
me Vr = n(h/2π)
Where me is the electron's mass, v is its velocity, r is the orbit radius, n is a positive integer representing the orbit number, and h is Planck's constant.
4. Radiation Absorption and Emission: Bohr's model explains atomic spectra, particularly the emission spectra of hydrogen, by suggesting that electrons emit or absorb energy in the form of electromagnetic radiation when they transition between different energy levels. The energy of the emitted or absorbed photon is equal to the energy difference between the initial and final states of the electron.
5. Stability of Orbits: Bohr's model proposed that certain orbits are more stable than others, corresponding to lower energy levels. Electrons tend to occupy the lowest energy orbit possible, known as the ground state. When atoms absorb energy, electrons can move to higher energy orbits, but they typically return to lower energy orbits, emitting the excess energy as electromagnetic radiation.
The frequency of the radiation emitted or absorbed during a transition between two orbits is determined by the energy difference between those orbits, following Bohr's frequency rule:
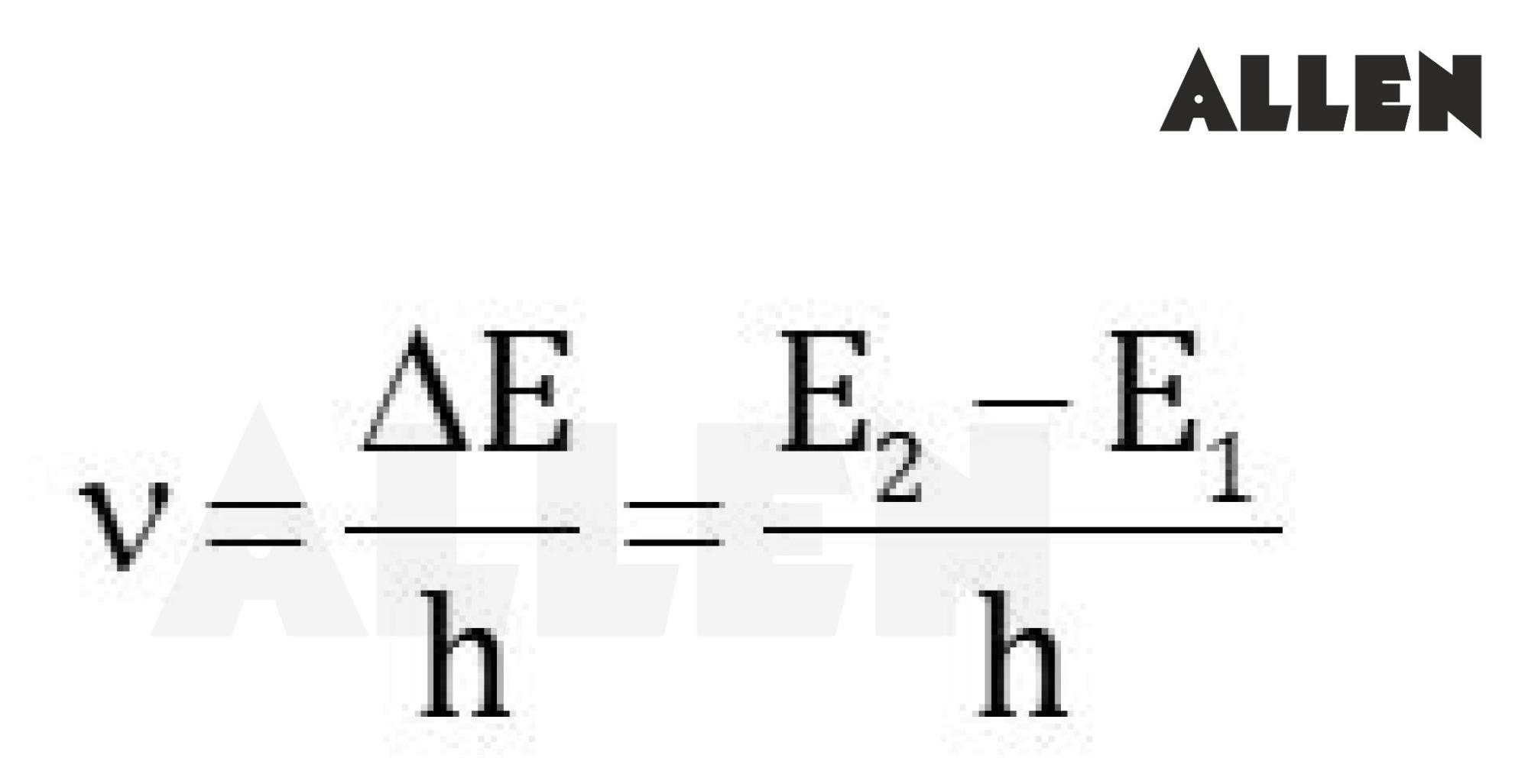
Where E1 and E2 are the energies of the lower and higher allowed energy states respectively. This expression is commonly known as Bohr's frequency rule.
The angular momentum of an electron in a given stationary state can be expressed as in equation.
me Vr = n(h/2π)
Thus, an electron can move only in those orbits for which its angular momentum is integral multiple of h/2π , that is why only certain fixed orbits are allowed.
Applications of Bohr's Atomic Model
- Explanation of Atomic Spectra: Bohr's model explained the discrete line spectra observed in the emission and absorption of light by atoms.
- Quantum Mechanics Development: It played an important role in the development of quantum mechanics by introducing the idea of quantized energy levels in atomic systems.
- Prediction of Atomic Properties: The model made predictions about atomic properties, such as the existence of spectral series in hydrogen, which were later confirmed by experiments.
- Stimulation of Atomic Physics Research: Bohr's model stimulated further research in atomic physics, leading to advancements in spectroscopy, quantum optics, and atomic spectroscopy.
- Technological Implications: While not directly leading to technological applications, Bohr's model laid the groundwork for technologies such as lasers, semiconductors, and nuclear energy by providing insights into atomic structure and behavior.
Limitations of the Bohr's Model
(1) Bohr's theory does not explain the spectrum of multi electron atom/ion.
(2) Why the Angular momentum of the revolving electron is equal to , has not been explained by Bohr's theory.
(3) Bohr inter related quantum theory of radiation and classical laws of physics without any theoretical explanation.
(4) Bohr's theory does not explain the fine structure of the spectral lines. Fine structure of the spectral line is obtained when the spectrum is viewed by a spectroscope of more resolution power.
(5) Bohr's theory does not explain the splitting of spectral lines in the presence of magnetic field (Zeeman's effect) or electric field (Stark effect)
The limitations of Bohr's atomic model led scientists to search for a better understanding of atoms.Two fundamental breakthroughs set the stage for this exploration:
- Dual behavior of matter: Scientists discovered that tiny particles like electrons sometimes act like waves and sometimes like particles. This duality challenged traditional ideas and hinted at a need for a more flexible model.
- Heisenberg uncertainty principle: This principle states that we can't know both the exact position and speed of particles simultaneously. It highlighted the inherent uncertainty in the behavior of particles at the atomic level.
5.0Development of Quantum Mechanical Model
These developments lead to the creation of the quantum mechanical model of the atom, which provided a more accurate and versatile description of atomic behavior.
Quantum mechanics, a theory developed independently by Werner Heisenberg and Erwin Schrödinger in 1926, explores the laws of motion for microscopic objects. It aligns with classical results when applied to macroscopic objects.
Table Of Contents
- 1.0What is Atomic Structure?
- 2.0Atomic Models
- 2.1Thomson's Plum Pudding Model (1898)
- 2.2Rutherford's Nuclear Model (1911)
- 3.0Development of Leading Model of Bohr
- 4.0Bohr's Atomic Model
- 4.1Applications of Bohr's Atomic Model
- 4.2Limitations of the Bohr's Model
- 5.0Development of Quantum Mechanical Model
Frequently Asked Questions:
Atomic structure refers to the arrangement of subatomic particles -protons, neutrons, and electrons in an atom. Protons and neutrons are located in the nucleus at the center, while electrons orbit the nucleus in shells.
Quantum mechanics is a fundamental theory in physics that provides a description of the physical properties of nature at the scale of atoms and subatomic particles. It introduces concepts like wave-particle duality, the uncertainty principle, and the probabilistic nature of particle properties.
The Bohr model treats electrons as particles in fixed orbits around the nucleus, while quantum mechanics describes electrons as wave-like distributions that exist in regions called orbitals. Unlike Bohr's orbits, quantum mechanical orbitals define probabilities of finding an electron in a particular location.
Join ALLEN!
(Session 2025 - 26)