Cholesterol Synthesis Pathway
It is a vital substance found in every cell of the human body, essential for maintaining overall health and proper physiological functions. It is a waxy, fat-like compound crucial in various biological processes. Although often associated with negative health outcomes due to its link to cardiovascular diseases, cholesterol is indispensable for the body.
1.0Cholesterol Synthesis
Cholesterol is widely recognized, primarily because of its association with increased risk of cardiovascular diseases due to high blood levels. However, its crucial roles are often overlooked. Cholesterol is essential for maintaining cellular membrane integrity and serves as a precursor for the synthesis of steroid hormones and bile acids. While cholesterol is vital for many animals, including humans, it does not need to be consumed through the diet since it can be synthesized by all cells from simple precursors. Despite its complex 27-carbon structure, all the carbon atoms in cholesterol originate from a single precursor: acetate.
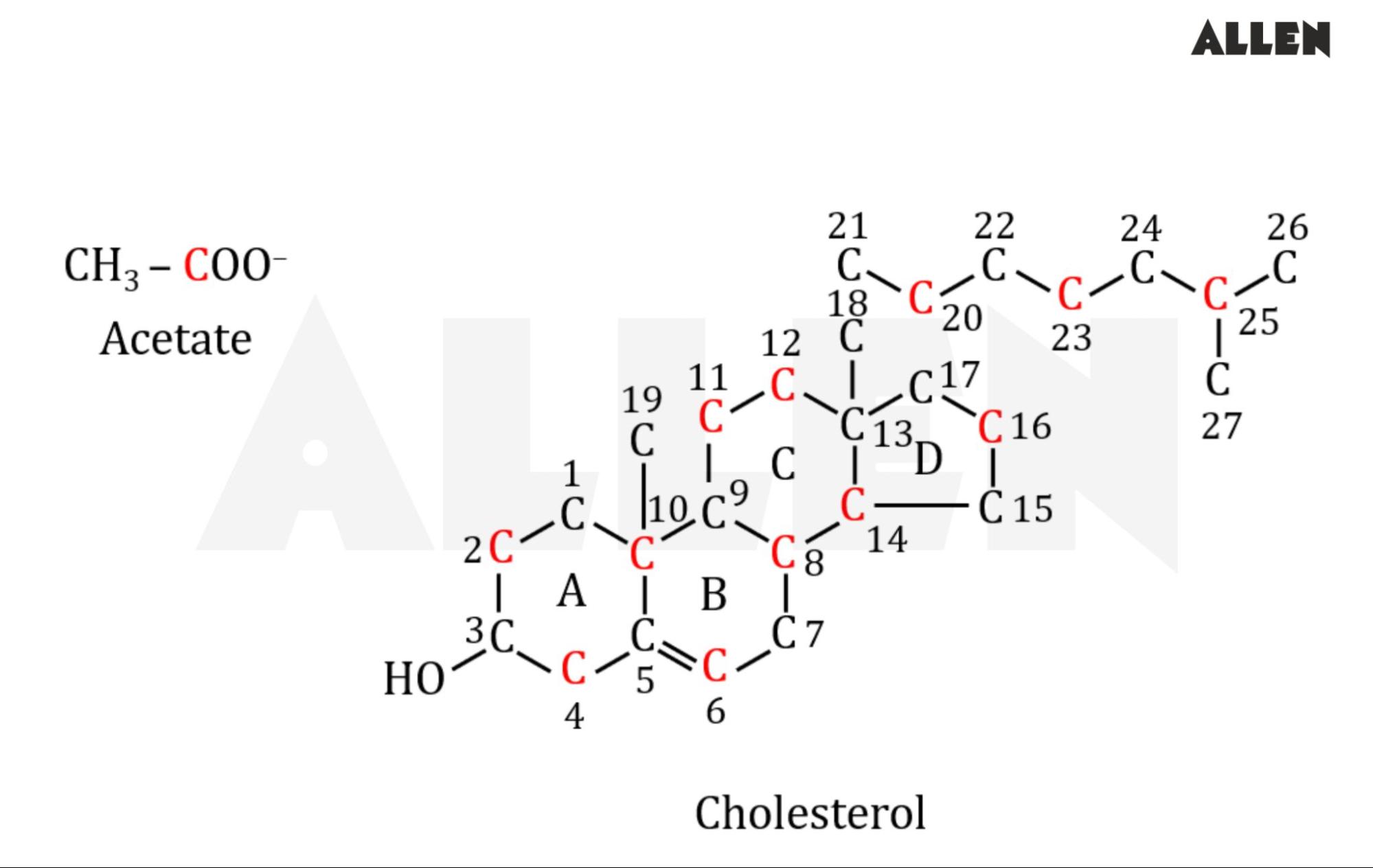
2.0What is Cholesterol Synthesis Pathway?
Cholesterol Is Made from Acetyl-CoA in Four Stages
Cholesterol, like long-chain fatty acids, is made from acetyl-CoA. Synthesis takes place in four stages:
1. Condensation of three acetate units to form a six-carbon intermediate, mevalonate;
2. Conversion of mevalonate to activated isoprene units;
3. Polymerization of six 5-carbon isoprene units to form the 30-carbon linear squalene; and
4. Cyclization of squalene forms the four rings of the steroid nucleus, followed by a series of additional modifications, including oxidations and the removal or migration of methyl groups, to produce cholesterol.
3.0Steps of Cholesterol Synthesis
Stage1: Synthesis of Mevalonate from Acetate
The initial stage of cholesterol biosynthesis produces the intermediate mevalonate. This begins with the condensation of two acetyl-CoA molecules to form acetoacetyl-CoA. Acetoacetyl-CoA then combines with a third acetyl-CoA molecule to produce the six-carbon compound β-hydroxy-β-methylglutaryl-CoA (HMG-CoA). These two reactions are catalyzed by the enzymes thiolase and HMG-CoA synthase, respectively. It’s important to note that the cytosolic HMG-CoA synthase involved in cholesterol synthesis is different from the mitochondrial isozyme that facilitates HMG-CoA synthesis during ketone body formation. The crucial and rate-limiting step in this pathway is the reduction of HMG-CoA to mevalonate, a reaction that requires two NADPH molecules to donate electrons. HMG-CoA reductase, an integral membrane protein located in the smooth endoplasmic reticulum (ER), is the key regulatory enzyme in cholesterol biosynthesis.
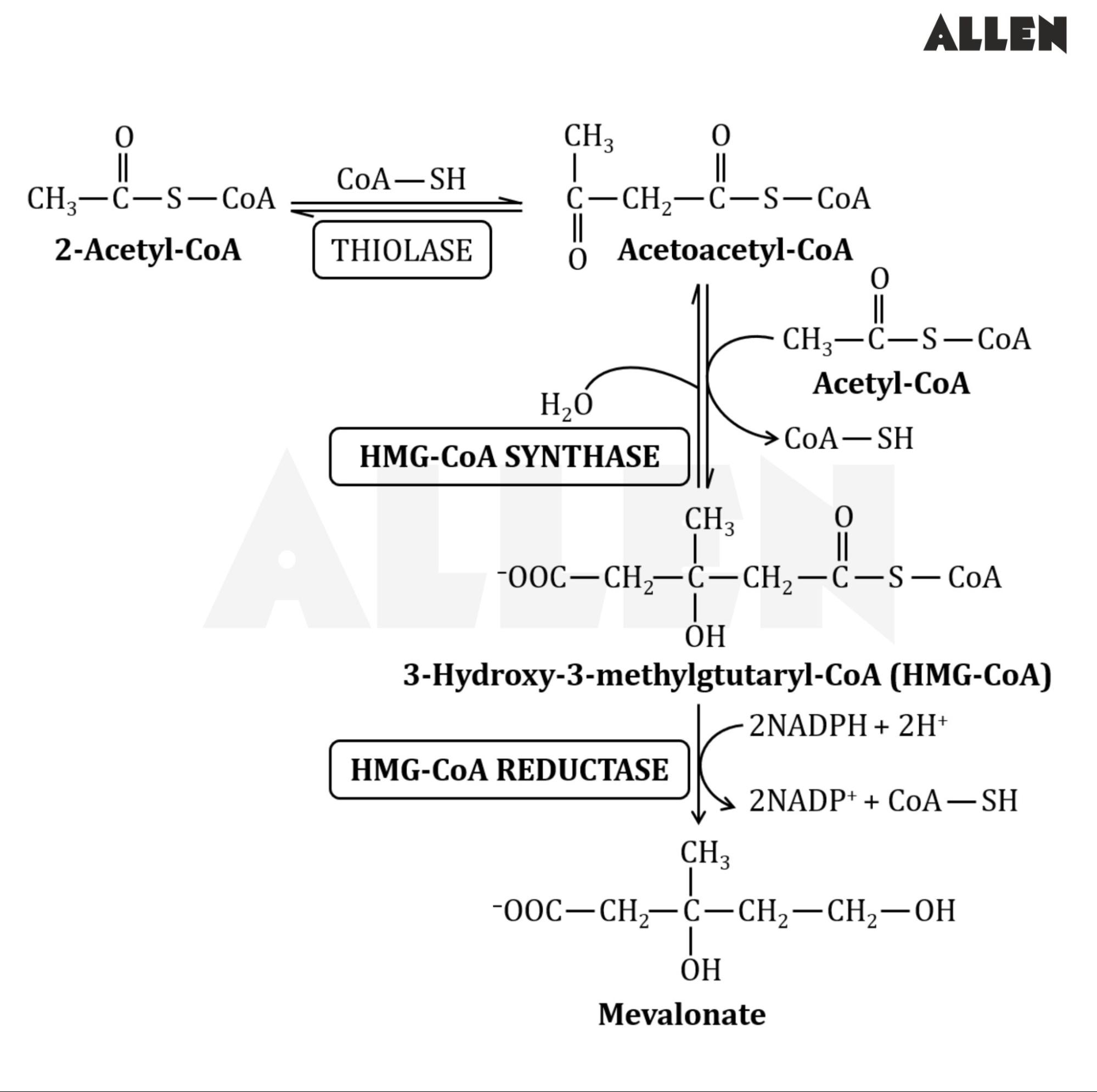
Stage 2: Conversion of Mevalonate to Two Activated Isoprenes
In the subsequent stage of cholesterol synthesis, three phosphate groups are transferred from three ATP molecules to mevalonate, forming 3-phospho-5-pyro phosphomevalonate. The phosphate attached to the C-3 hydroxyl group of mevalonate, along with a nearby carboxyl group, are cleaved off, resulting in the formation of a double bond in the five-carbon molecule ∆3-isopentenyl pyrophosphate. This molecule represents the first of two activated isoprenes essential for cholesterol synthesis. The isomerization of ∆3-isopentenyl pyrophosphate produces the second activated isoprene, dimethylallyl pyrophosphate.
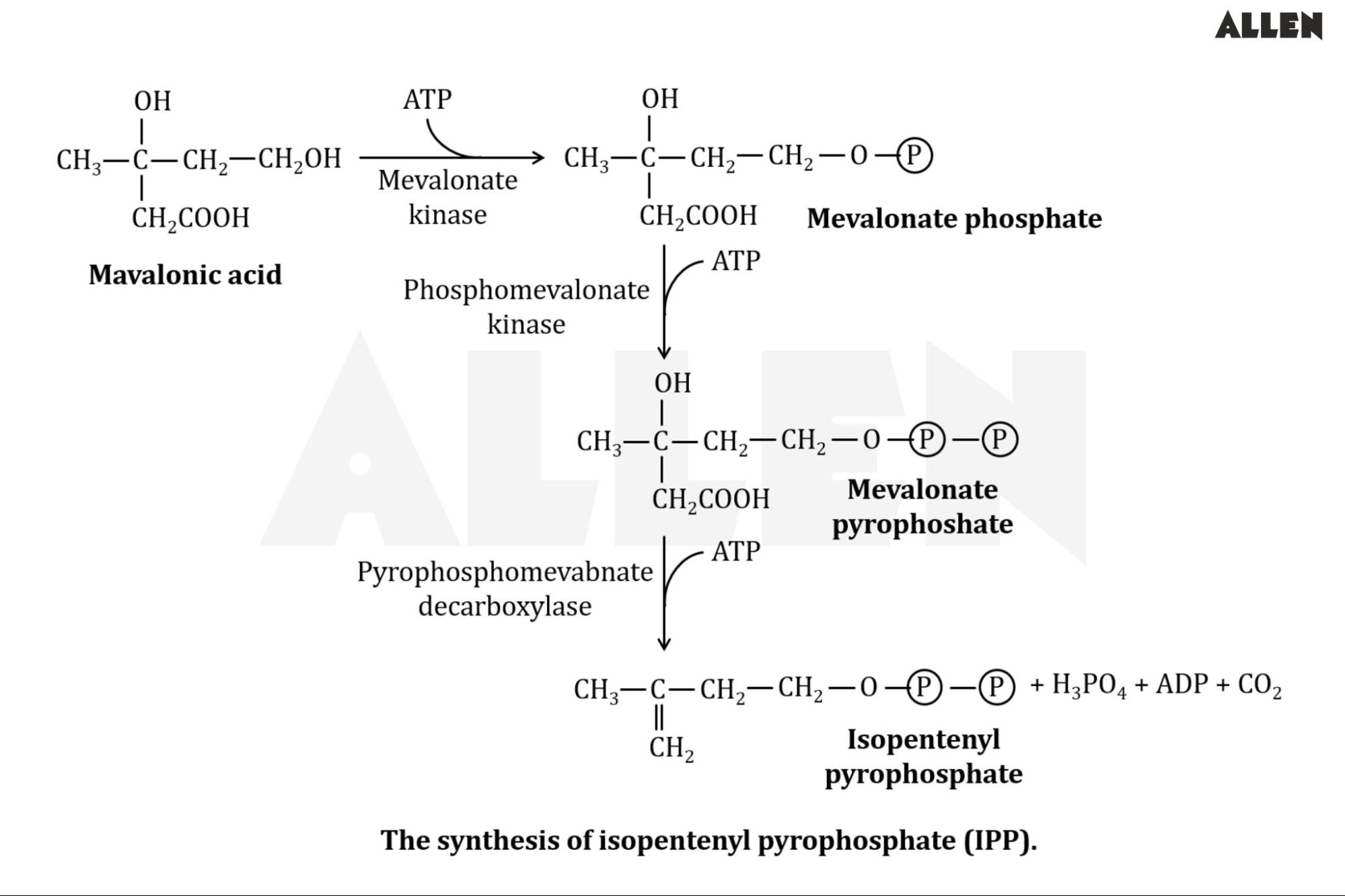
Stage 3: Condensation of Six Activated Isoprene Units to Form Squalene
Isopentenyl pyrophosphate and dimethylallyl pyrophosphate first undergo a head-to-tail condensation, resulting in the formation of a 10-carbon chain known as geranyl pyrophosphate, with one pyrophosphate group being displaced. Geranyl pyrophosphate then undergoes another head-to-tail condensation with isopentenyl pyrophosphate, producing a 15-carbon intermediate called farnesyl pyrophosphate. Lastly, two molecules of farnesyl pyrophosphate join in a head-to-head condensation, eliminating both pyrophosphate groups, to form squalene.
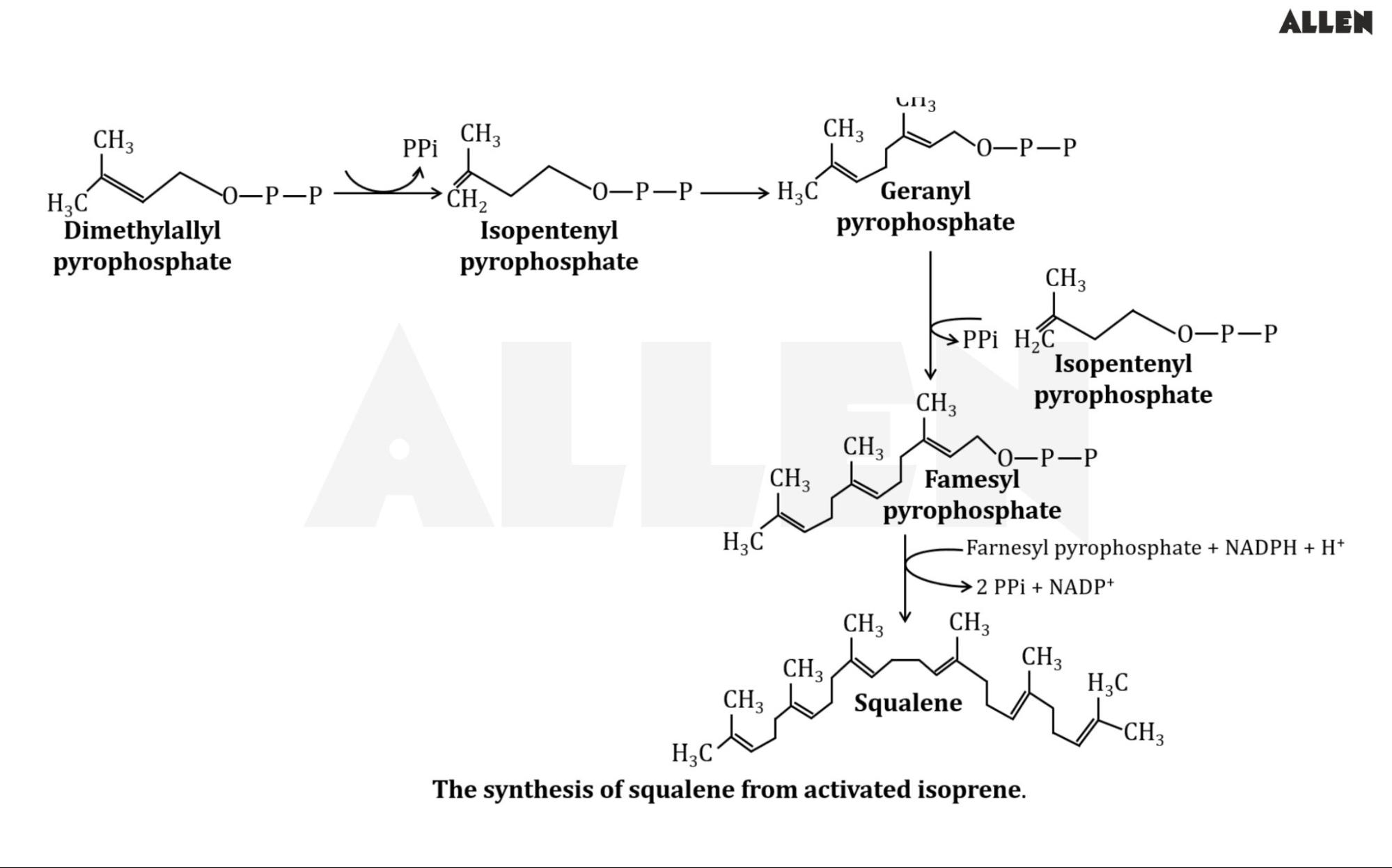
Stage 4: Conversion of Squalene to the Four-Ring Steroid Nucleus
All sterols share a common structure with four fused rings forming the steroid nucleus and all contain a hydroxyl group at carbon-3, which is why they are called "sterols." During the cholesterol biosynthesis process, squalene monooxygenase introduces one oxygen atom from O2 into the end of the squalene chain, producing squalene epoxide. This enzyme acts as a mixed-function oxidase, with NADPH reducing the remaining oxygen atom to water. The product, squalene 2,3-epoxide, has double bonds arranged in a way that allows it to undergo a significant concerted reaction, transforming the linear squalene epoxide into a cyclic structure. In animal cells, this cyclization results in the formation of lanosterol, which features the four-ring structure typical of the steroid nucleus. Lanosterol is then converted into cholesterol through a series of about 20 additional reactions, involving the movement and removal of various methyl groups.
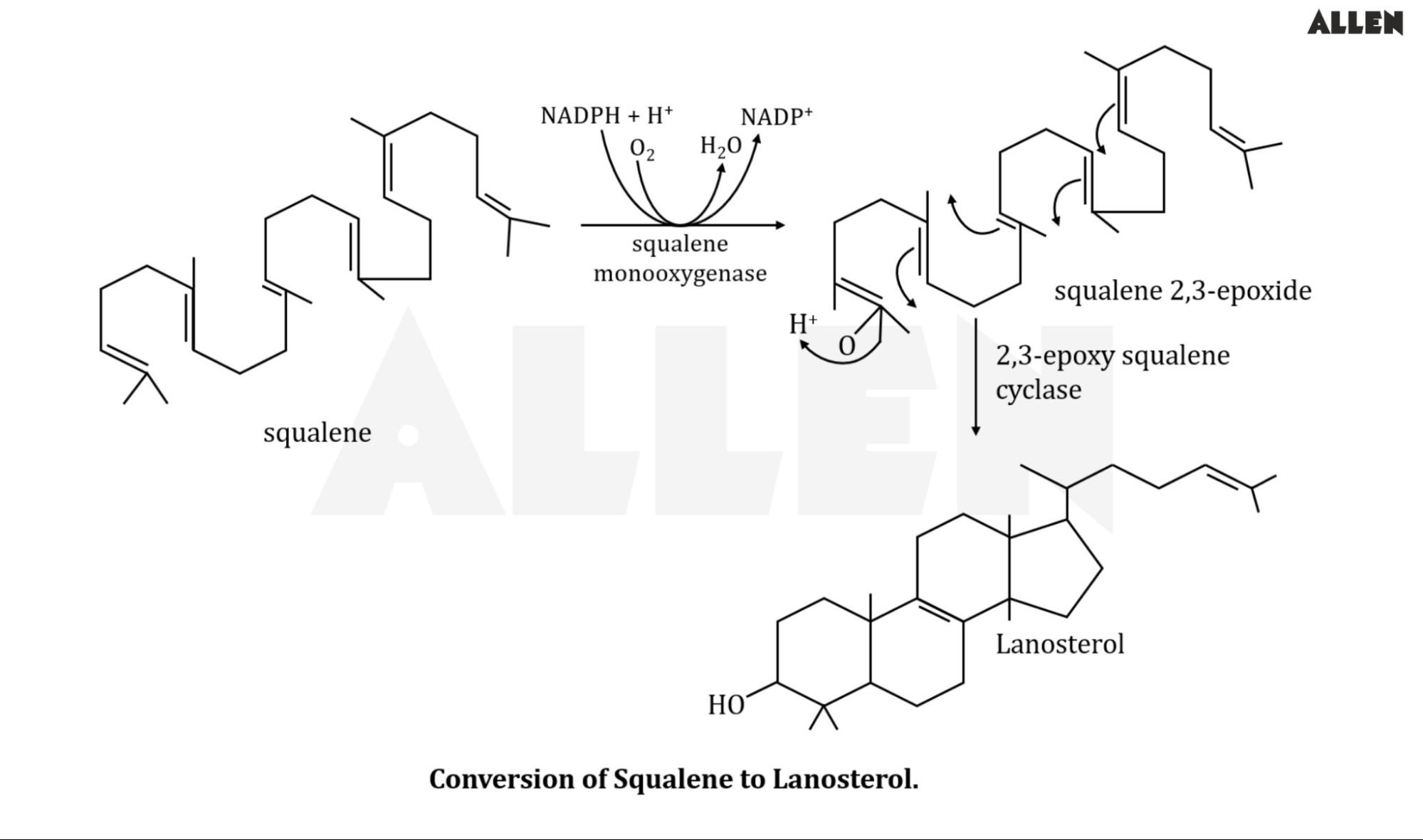
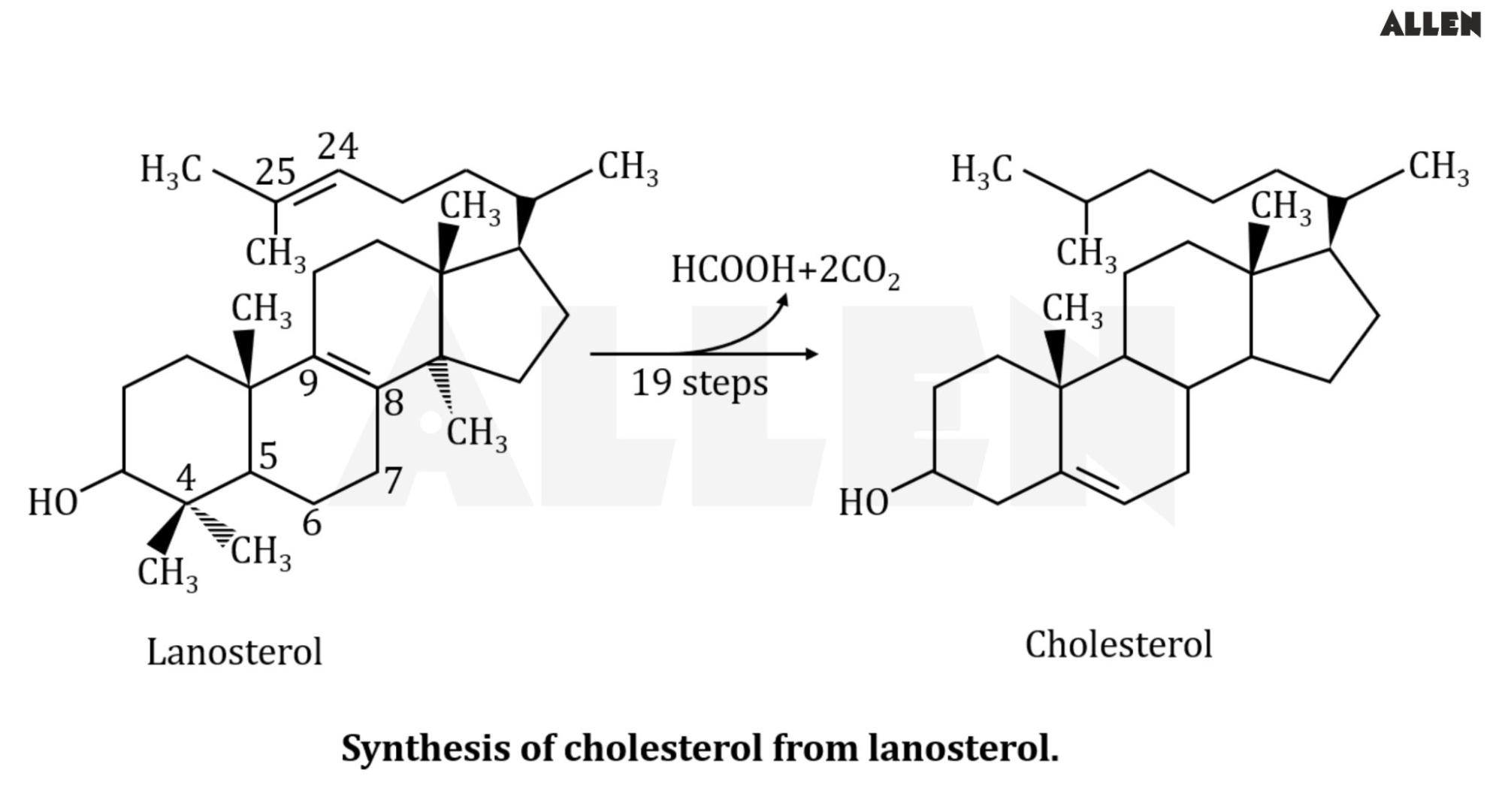
4.0Regulation of Cholesterol Synthesis
Cholesterol synthesis in the body is a highly regulated process that ensures a balance between the body's needs and the levels of cholesterol present. The regulation occurs primarily at the level of HMG-CoA reductase, the rate-limiting enzyme in the cholesterol synthesis pathway. Here are the key mechanisms involved in the regulation of cholesterol synthesis:
1. Feedback Inhibition
- Cholesterol Levels: High intracellular cholesterol levels inhibit the activity of HMG-CoA reductase, thereby reducing cholesterol synthesis. This negative feedback loop ensures that further production is decreased when sufficient cholesterol is available.
2. Hormonal Regulation
- Insulin: Insulin promotes the activity of HMG-CoA reductase, enhancing cholesterol synthesis. This is consistent with the body's anabolic state after food intake.
- Glucagon: Glucagon inhibits HMG-CoA reductase activity, reducing cholesterol synthesis during fasting or catabolic states.
- Thyroid Hormones: These hormones can also regulate HMG-CoA reductase, generally increasing its activity and promoting cholesterol synthesis.
3. Gene Expression
- SREBP (Sterol Regulatory Element-Binding Proteins): SREBPs are transcription factors that regulate the expression of genes involved in cholesterol and fatty acid synthesis, including the HMG-CoA reductase gene. When cholesterol levels are low, SREBPs are activated and move to the nucleus to increase the transcription of these genes, enhancing cholesterol synthesis.
- SCAP (SREBP Cleavage-Activating Protein): SCAP senses cholesterol levels in the endoplasmic reticulum and regulates the activation of SREBPs. When cholesterol is low, SCAP facilitates the transport of SREBPs to the Golgi apparatus, where they are activated.
4. Post-Translational Modification
- Phosphorylation: HMG-CoA reductase activity is modulated by phosphorylation and dephosphorylation. AMP-activated protein kinase (AMPK) phosphorylates and inactivates HMG-CoA reductase when cellular energy levels are low, reducing cholesterol synthesis.
- Dephosphorylation: Protein phosphatases dephosphorylate and activate HMG-CoA reductase when energy levels are adequate, promoting cholesterol synthesis.
5. Dietary Regulation
- Dietary Intake: Consumption of dietary cholesterol can suppress endogenous cholesterol synthesis. This is partly due to the absorption of dietary cholesterol and its incorporation into cellular cholesterol pools, which contributes to feedback inhibition.
- Fatty Acids: Saturated fats can increase LDL cholesterol levels, while unsaturated fats, particularly omega-3 fatty acids, can have the opposite effect.
6. Pharmacological Regulation
- Statins: Statin drugs are HMG-CoA reductase inhibitors used to lower cholesterol levels in patients with hypercholesterolemia. By inhibiting this key enzyme, statins effectively reduce the synthesis of cholesterol, leading to lower blood cholesterol levels.
Table of Contents
- 1.0Cholesterol Synthesis
- 2.0What is Cholesterol Synthesis Pathway?
- 3.0Steps of Cholesterol Synthesis
- 4.0Regulation of Cholesterol Synthesis
Frequently Asked Questions
Cholesterol synthesis occurs in the cytoplasm and the cell's endoplasmic reticulum (ER). The enzymes involved in the pathway are located in these cellular compartments.
SREBPs (Sterol Regulatory Element-Binding Proteins) are transcription factors that regulate the expression of genes involved in cholesterol and fatty acid synthesis. When cellular cholesterol levels are low, SREBPs are activated and move to the nucleus, increasing the transcription of these genes and thereby promoting cholesterol synthesis.
Hormones such as insulin and glucagon play significant roles in regulating cholesterol biosynthesis. Insulin promotes the activity of HMG-CoA reductase, enhancing cholesterol synthesis, while glucagon inhibits the enzyme, reducing synthesis.
HMG-CoA reductase is considered the key regulatory enzyme because it catalyzes the rate-limiting step of the pathway and is subject to multiple layers of regulation, including feedback inhibition, hormonal control, gene expression, and post-translational modifications. This central role makes it a crucial control point in maintaining cholesterol balance in the body.
Join ALLEN!
(Session 2025 - 26)