Krebs Cycle
Cellular respiration has three major stages. In the first, organic fuel molecules glucose, fatty acids, and some amino acids are oxidized to yield two-carbon fragments in the form of the acetyl group of acetyl-coenzyme A, or acetyl-CoA. In the second stage, the acetyl groups are fed into the citric acid cycle, oxidizing them to CO2; the energy released is conserved in the reduced electron carriers NADH and FADH2. In the third stage of respiration, these reduced coenzymes are themselves oxidized, giving up protons (H) and electrons. The electrons are transferred to O2 the final electron acceptor via a chain of electron carrying molecules known as the respiratory chain. This amount of energy is conserved during the process of electron transfer in the form of ATP through a process called oxidative phosphorylation.
1.0Introduction of Krebs Cycle
- The citric acid cycle is also known as the tricarboxylic acid (TCA) cycle or the Krebs cycle, in honour of Hans Krebs, whose research in the 1930s was responsible for elucidating this central metabolic process. Krebs was awarded the Nobel Prize in medicine in 1954 for his outstanding contribution.
- The krebs cycle occurs in the mitochondrial matrix. All the enzymes of TCA cycle, except Succinate dehydrogenase (in the inner mitochondrial membrane) present in matrix.
- During Kreb's cycle acetyl CoA is completely oxidised into CO2.
- Krebs cycle is also called Citric acid (CA) cycle because Ist Compound is Citric acid (6C). In this acid, 3 carboxylic groups (COOH) are found so process is also called TCA (Tricarboxylic Acid) cycle.
- In Kreb's cycle oxaloacetic acid (OAA) is the first member and it also act as first acceptor of Acetyl Co-A. In the end of this cycle OAA re-formed.
2.0Reactions of the Citric Acid Cycle
- Now we consider the oxidation of acetyl-CoA. This is accomplished by the first cyclic pathway we have encountered, known as the citric acid cycle. A cycle turn gets underway when the acetyl group of acetyl-CoA is transferred to a four-carbon compound called oxaloacetate to produce the six-carbon compound known as citrate. Citrate is transformed into isocitrate, also a six-carbon molecule, which is dehydrogenated with loss of CO2 to form the five-carbon compound alpha-ketoglutarate also known as oxoglutarate. Alpha-Ketoglutarate loses a second molecule of CO2 and ultimately yields the four-carbon compound succinate. Succinate is then enzymatically transformed in three steps into the four-carbon oxaloacetate which is then ready to react with another molecule of acetyl-CoA.
- In each turn, one acetyl group-the two carbons of the acetyl group-enter as acetyl-CoA, and two molecules of CO2 leave; one molecule of oxaloacetate is used in making citrate and one molecule of oxaloacetate is regenerated. No net removal of oxaloacetate is involved; it is theoretically possible for one molecule of oxalo-acetate to participate in oxidation of an infinite number of acetyl groups and, in actual fact, oxaloacetate occurs in cells in very low concentrations. Four of the eight steps are oxidations, whereby the energy of oxidation is almost completely conserved in the reduced coenzymes NADH and FADH2.
- As discussed earlier, although the citric acid cycle is central to energy-yielding metabolism, its role is not strictly energy conservation. Four and five carbon intermediates of the cycle are precursors for a wide variety of products. To replace intermediates removed for this purpose, cells use anaplerotic (replenishing) reactions, described below.
- In 1948, Eugene Kennedy and Albert Lehninger showed that, in eukaryotes, all of the reactions of the citric acid cycle take place within the mitochondria. Isolated mitochondria contained not only all the enzymes and coenzymes required for the citric acid cycle but also all the enzymes and proteins necessary for the last step of respiration, electron transfer, and ATP synthesis by oxidative phosphorylation.
- Mitochondria also contain the enzymes for the oxidation of fatty acids and some amino acids to acetyl-CoA, and the oxidative degradation of other amino acids to α-ketoglutarate, succinyl-CoA, or oxaloacetate. Thus, in non photosynthetic eukaryotes, the mitochondrion is the site of most energy-yielding oxidative reactions and of the coupled synthesis of ATP. In photosynthetic eukaryotes, mitochondria are the major site of ATP production in the dark, but in daylight chloroplasts produce most of the organism's ATP. In most prokaryotes, the enzymes of the citric acid cycle are in the cytosol, and the plasma membrane plays a role analogous to that of the inner mitochondrial membrane in ATP synthesis.
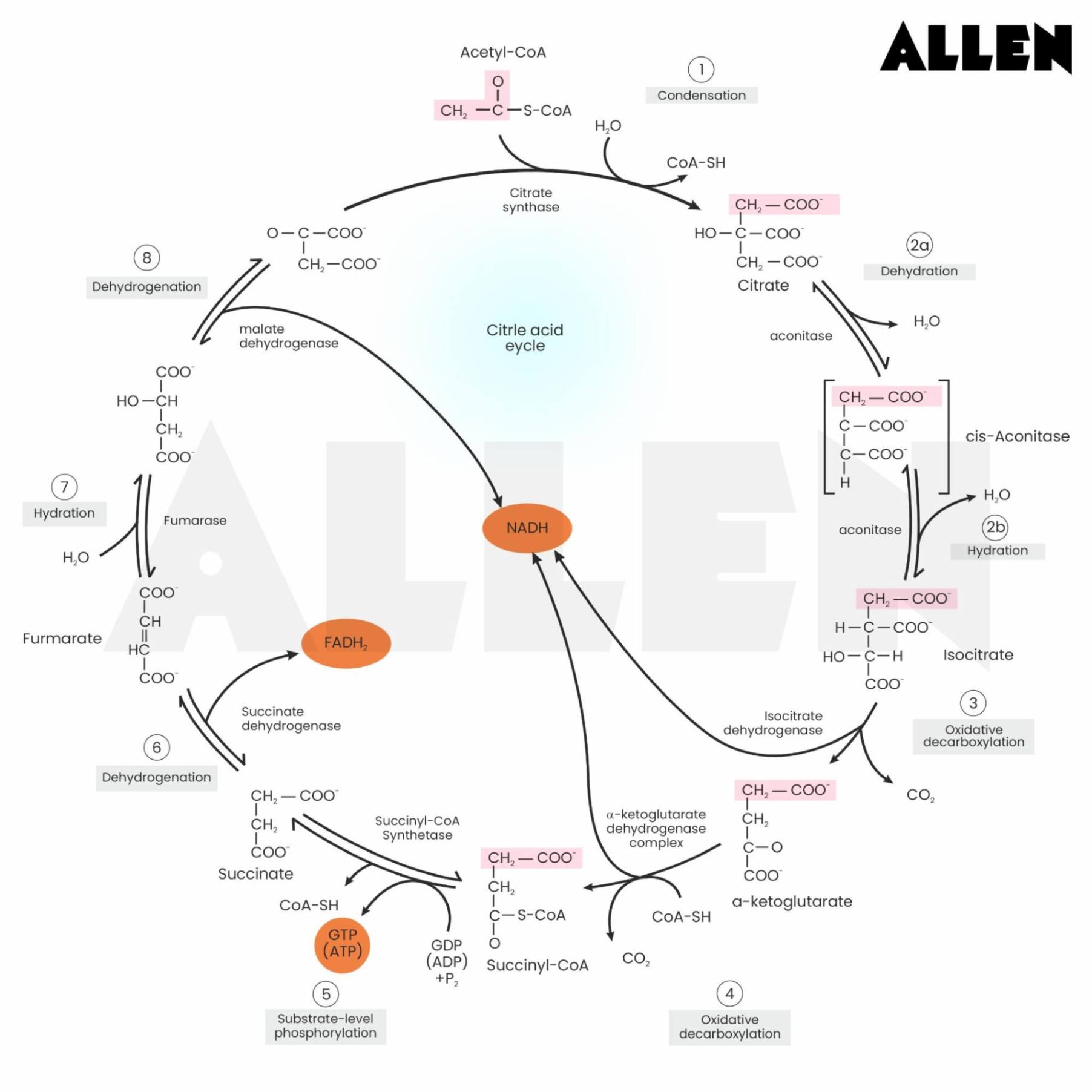
3.0The Citric Acid Cycle Has Eight Steps
In analyzing the eight successive steps of reactions in the cycle, special emphasis is given to special attention to the chemical changes that occur as citrate synthesized from acetyl-CoA and oxaloacetate is oxidized to produce CO2 and the energy of this oxidation is conserved in the reduced coenzymes NADH and FADH2.
(i) Formation of Citrate :
- The first reaction of the cycle is the condensation of acetyl-CoA with oxaloacetate to form citrate, catalyzed by citrate synthase:
- In this reaction the methyl carbon of the acetyl group is joined to the carbonyl group (C-2) of oxaloacetate. Citroyl-CoA is a transient intermediate formed on the active site of the enzyme. It rapidly undergoes hydrolysis to free CoA and citrate, which are released from the active site. The hydrolysis of this high energy thioester intermediate makes the forward reaction highly exergonic
(ii) Formation of Isocitrate via cis-Aconitate :
- The enzyme aconitase more formally, aconitate hydratase catalyzes the reversible transformation of citrate to isocitrate, through the intermediary formation of the tricarboxylic acid cis -aconitate, which normally does not dissociate from the active site. Aconitase can promote the reversible addition of H2O to the double bond of enzyme bound cis-aconitate in two different ways, one leading to citrate and the other to isocitrate:
- Although less than 10% isocitrate remains in the equilibrium mixture at pH 7.4 and 25 °C, the cell favors the right reaction because the next step in the cycle quickly depletes the isocitrate, driving its steady-state concentration down. The iron-sulfur center within aconitase serves a dual purpose-together in binding substrate at the active site and in catalytically adding or removing H2O.
(iii) Oxidation of Isocitrate to alpha-Ketoglutarate and CO2 :
- Isocitrate dehydrogenase is the enzyme that catalyzes the next step in which isocitrate undergoes oxidative decarboxylation to form alpha-ketoglutarate. Mn2+ in the active site interacts with the carbonyl group of the intermediate oxalosuccinate, formed transiently but not leaving the binding site until decarboxylation has converted it to alpha-ketoglutarate. Mn2+ also stabilizes the enol that forms transiently by decarboxylation.
(iv) Oxidation of alpha-Ketoglutarate to Succinyl-CoA and CO2 :
- Another oxidative decarboxylation follows, which converts α-ketoglutarate to succinyl-CoA and CO2, all done by the action of the alpha-ketoglutarate dehydrogenase complex, with NAD as electron acceptor and CoA as the carrier of the succinyl group. The energy of oxidation of α-ketoglutarate is conserved in the formation of the thioester bond of succinyl-CoA:
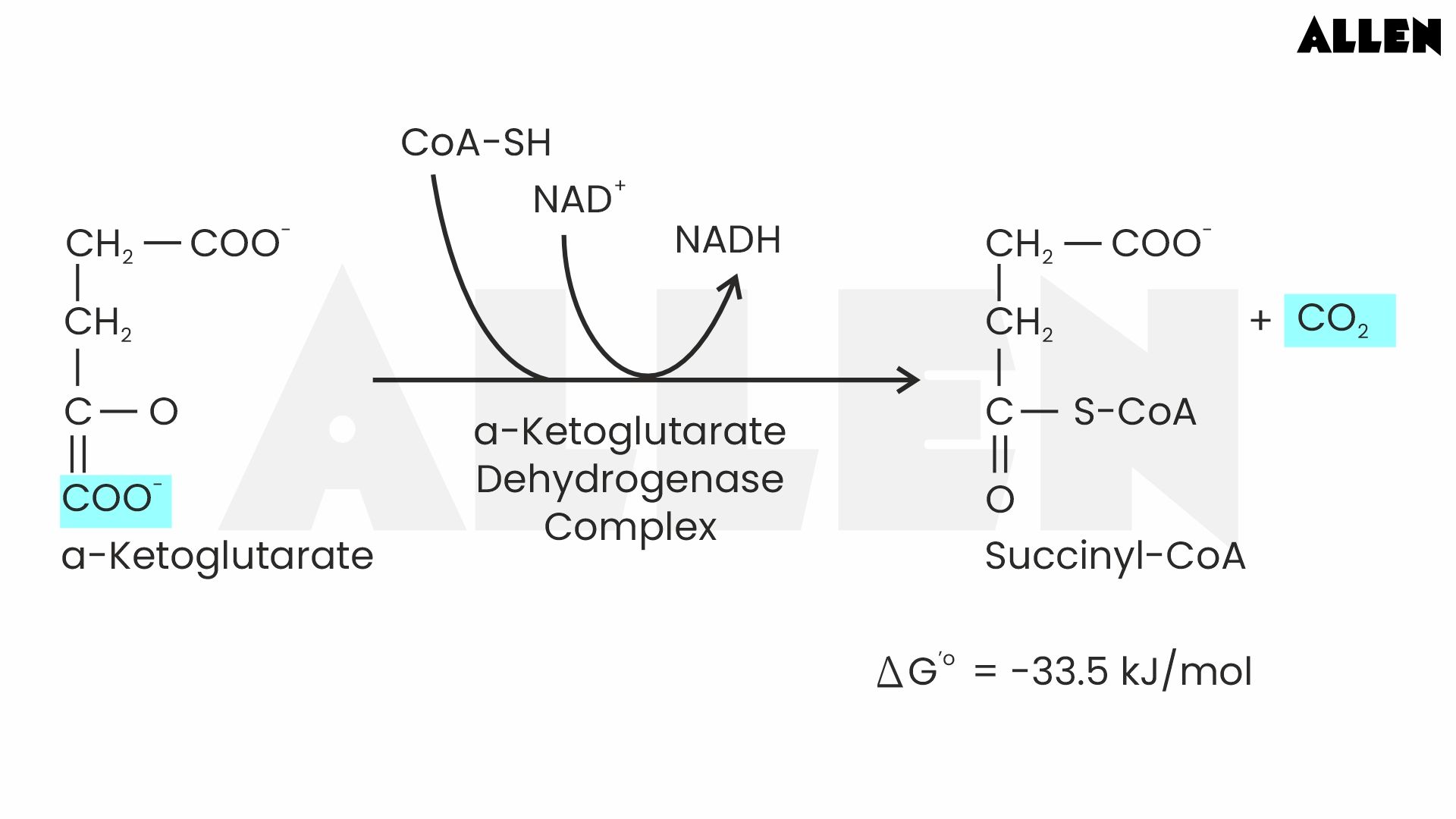
- This reaction is very similar to the pyruvate dehydrogenase reaction, and the α-ketoglutarate dehydrogenase complex is quite similar in structure and function to the PDH complex. It contains three enzymes, just like E1, E2, and E3 of the PDH complex, as well as enzyme-bound TPP, bound lipoate, FAD, NAD, and coenzyme A.
- Both complexes are certainly derivatives of some common evolutionary ancestor. The E1 components of the two complexes are similar in structure although their amino acid sequences are different and, obviously, their binding specificities differ: E1 of the PDH complex binds pyruvate, while E1 of the α-ketoglutarate dehydrogenase complex binds αalpha-ketoglutarate. In the two complexes, the E2 components are very similar, both having covalently bound lipoyl moieties. The subunits of E3 are identical in the two enzyme complexes.
(v) Conversion of Succinyl-CoA to Succinate :
- Succinyl-CoA, like acetyl-CoA, carries its energy in the form of a thioester bond, whose hydrolysis has a strongly negative standard free energy. In the next step of the citric acid cycle, energy released in the breakage of this bond is used to drive the synthesis of a phosphoanhydride bond in either GTP or ATP, with a net G of only 2.9 kJ/mol. In the process, succinate is formed:
- The GTP formed by succinyl- CoA synthetase can donate its terminal phosphoryl group to ADP to form ATP, in a reversible reaction catalyzed by nucleoside diphosphate kinase
(vi) Oxidation of Succinate to Fumarate :
- The succinate formed from succinyl-CoA is oxidized to fumarate by the flavoprotein succinate dehydrogenase:
- In eukaryotes, the succinate dehydrogenase is tightly bound to the inner mitochondrial membrane; in prokaryotes, to the plasma membrane. The enzyme contains three different iron-sulfur clusters and one molecule of covalently bound FAD. Electrons pass from succinate through the FAD and iron-sulfur centers before entering the chain of electron carriers in the mitochondrial inner membrane (or the plasma membrane in bacteria). This electron flow from succinate to the final electron acceptor, O2, is accompanied by the synthesis of approximately 1.5 molecules of ATP per pair of electrons (respiration-linked phosphorylation).
- Malonate is an analog of succinate and is not normally present in cells; it is a strong competitive inhibitor of succinate dehydrogenase and so blocks the activity of the citric acid cycle when added to mitochondria.
(vii) Hydration of Fumarate to Malate :
- The reversible hydration of fumarate to L-malate is catalyzed by fumarase (formally, fumarate hydratase). The transition state in this reaction is a carbanion:
(viii) Oxidation of Malate to Oxaloacetate :
- In the last reaction of the citric acid cycle, NAD-linked L-malate dehydrogenase catalyzes the oxidation of L-malate to Oxaloacetate
4.0Reactant and Product of Kreb’s cycle : per acetyl coenzyme A
5.0Summary of Krebs Cycle
- The citric acid cycle begins with the condensation of a two-carbon acetyl group with the four-carbon oxaloacetate to form a molecule of the six-carbon citrate. The acetyl group is completely oxidised into two molecules of CO2.
- The cycle includes four oxidations, which yield NADH2 at three steps and FADH2 at one step. One molecule of ATP is formed by substrate-level phosphorylation. Finally, the oxaloacetate is regenerated, which allows the cycle to continue.